Techniques Integrative Cardiac Bioelectricity Group
Microelectrode arrays (MEAs)
Multiple Site Recording of Extracellular Potential in Cardiac Cell Cultures
Cardiac function relies on the coordinated propagation of the action potential (AP), the rhythmic electrical signal that triggers the contraction of every cardiac cell. Arrhythmias are frequent complications of heart disease and can potentiate heart failure, lead to stroke and cause sudden death. The AP is an intricate dynamic phenomenon, which relies on the function of ion channels. The complexity of the AP and of cardiac conduction explains why the prevention and treatment of arrhythmias still remains a great challenge.
Our research addresses cardiac conduction with an interdisciplinary approach unifying in vitro experiments and computer simulations. In vitro, we use a custom developed system to stimulate and record the electrical activity of cardiac cell cultures with microelectrode arrays. This approach is combined with techniques to pattern the cultures to predefined geometries. In computer simulations, we reconstruct conduction using mathematical models of the cardiac cell. These simulations provide insights into aspects not accessible experimentally.
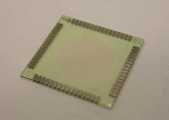
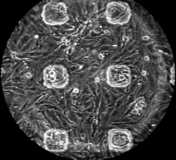
The arrays are mounted in a custom built amplificator setup, which permits the recording of extracellular action potentials from the culture during extended periods of time and under incubating conditions. Using photolithographic techniques, it is also possible to pattern the growth of the cultures to predefined geometries. This opens the possibility to study the effects of tissue architecture on the conduction of the cardiac action potential.
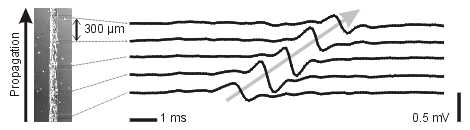
Stretchable microelectrode arrays
We recently developed an unprecedented platform based on stretchable microelectrode arrays (sMEAs) permitting to investigate the effects of accurately controlled strain on electrophysiological properties of cardiac cell cultures. The sMEAs were developed in collaboration with the group of Prof. S. Lacour (Laboratory for Soft Bioelectronic Interfaces, Swiss Federal Institute of Technology, EPFL, Geneva).
The platform integrates these sMEAs into a custom-made stretching system permitting to stretch the sMEAs along two perpendicular axes. An image acquisition and processing system allows accurately measuring and adjusting strain. This new system thus permits the application of controlled deformations to cultures of cardiac cells while recording their electrical activity.
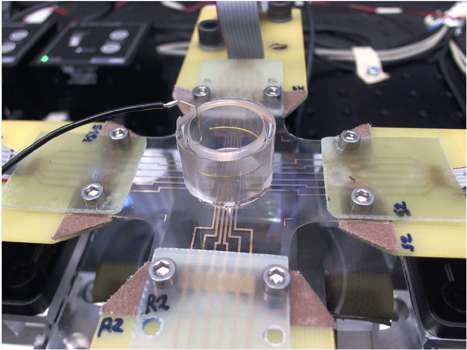
Modeling
Mathematical Modeling of Conduction
We use mathematical models of the cardiac cell (e.g., the Luo-Rudy model) to reconstruct conduction in cell networks.
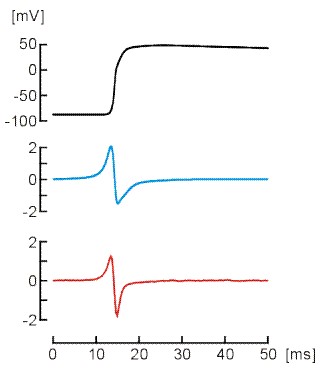
Mathematical Modeling of Ephaptic Coupling
We develop two- and three- dimensional finite element modeling frameworks of cardiac intercalated discs to examine the role of ephaptic coupling in action potential propagation.