Projects Integrative Cardiac Bioelectricity Group
Introduction and background
Cardiac function relies on the coordinated propagation of the action potential (AP), the rhythmic electrical signal that triggers the contraction of every cardiac cell. Arrhythmias are frequent complications of heart disease and can potentiate heart failure, lead to stroke and cause sudden death. The AP is an intricate dynamic phenomenon, which relies on the function of ion channels. The complexity of the AP and of cardiac conduction explains why the prevention and treatment of arrhythmias still remains a great challenge.
Our research addresses cardiac conduction with an interdisciplinary approach unifying in vitro experiments and computer simulations. In vitro, we use a custom developed system to stimulate and record the electrical activity of cardiac cell cultures with microelectrode arrays. This approach is combined with techniques to pattern the cultures to predefined geometries. In computer simulations, we reconstruct conduction using mathematical models of the cardiac cell. These simulations provide insights into aspects not accessible experimentally.
Current Projects
Effects of cardiac tissue deformation on its electrophysiological properties
The pumping heart continuously changes shape during every beat and the electrophysiological properties of cardiac tissue are influenced by deformation (strain). This so-called mechano-electrical feedback involves numerous factors such as stretch-activated channels, modulation of ion channel function as well as changes in tissue resistance and, possibly, capacitance.
Using cardiac cell cultures grown on our recently developed stretchable electrode arrays (collaboration with Prof. S. Lacour, Laboratory for Soft Bioelectronic Interfaces of the EPFL), we are investigating the effects of strain on the electrical activity of cardiac cell cultures. We demonstrated that uniaxial strain applied in the direction of action potential propagation exerts a different effect on conduction velocity compared to uniaxial strain applied perpendicular to propagation. This difference is explained by the different effects of these two types of strain on myoplasmic resistance.
Publications

Uniaxial strain of cultured mouse and rat cardiomyocyte strands slows conduction more when its axis is parallel to impulse propagation than when it is perpendicular. Acta Physiologica 223:e13026, 2018 DOI pdf
Ephaptic conduction in cardiac tissue
Classically, cardiac conduction relies on the Na+ current (INa) flowing through voltage-gated Na+ channels during excitation and on intercellular currents through gap junctions. However, an alternate mechanism has been proposed for impulse propagation, especially when gap junctional coupling is reduced. This mechanism, called «ephaptic conduction» or «ephaptic coupling», is mediated by large negative extracellular potentials occurring in intercalated discs, i.e., in the narrow extracellular clefts separating adjacent cardiac cells (Figure). Activation of Na+ channels in the membrane on one side of the intercalated disc causes a large INa and thus a large negative extracellular potential (Ve) in the cleft, which translates, on the other side, into membrane depolarization and Na+ channel activation. This controversial mechanism is the object of our research. In collaboration with the team of Prof. H. Abriel, we demonstrated that confining the extracellular space near a cell expressing human cardiac sodium channels affects the sodium current as predicted by computer simulations taking the ephaptic mechanism into account. Furthermore, our computer simulations demonstrated that the clustering of Na+ channels in the intercalated disc potentiates ephaptic conduction. Na+ channels preferentially cluster in perinexi, which are nanodomains that surround gap junctions. In a recent study, we showed that these narrow perinexi are privileged sites for ephaptic coupling.
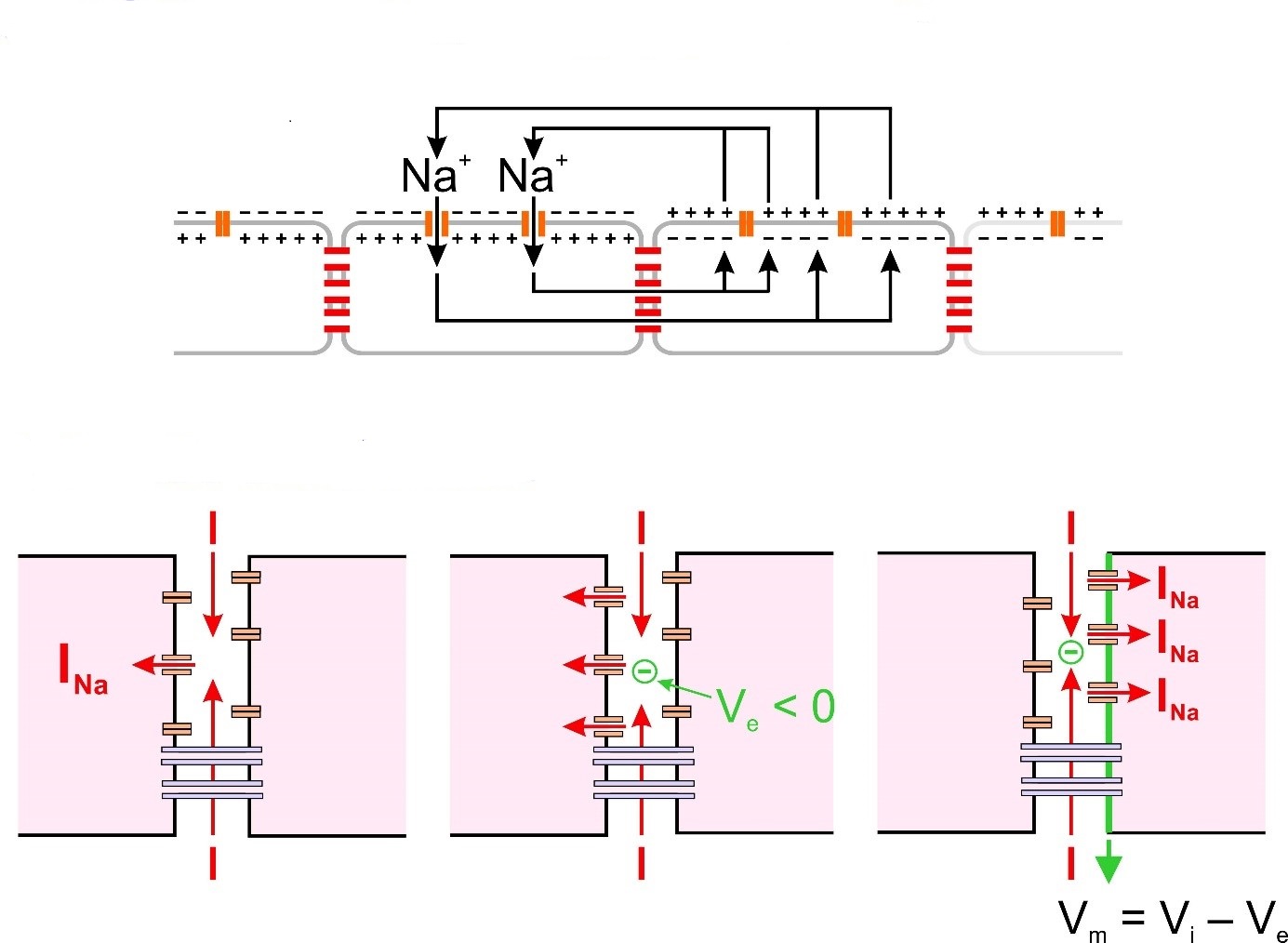
Classical vs. ephaptic conduction.
Top: The classical mechanism, based on intercellular current flow through gap junctions.
Bottom: The ephaptic mechanism, based on large negative extracellular potentials occurring in narrow intercellular clefts in the intercalated discs.
Publications
Localization of sodium channels in intercalated disks modulates cardiac conduction. Circ. Res. 91, No 12, 1176-1182, 2002
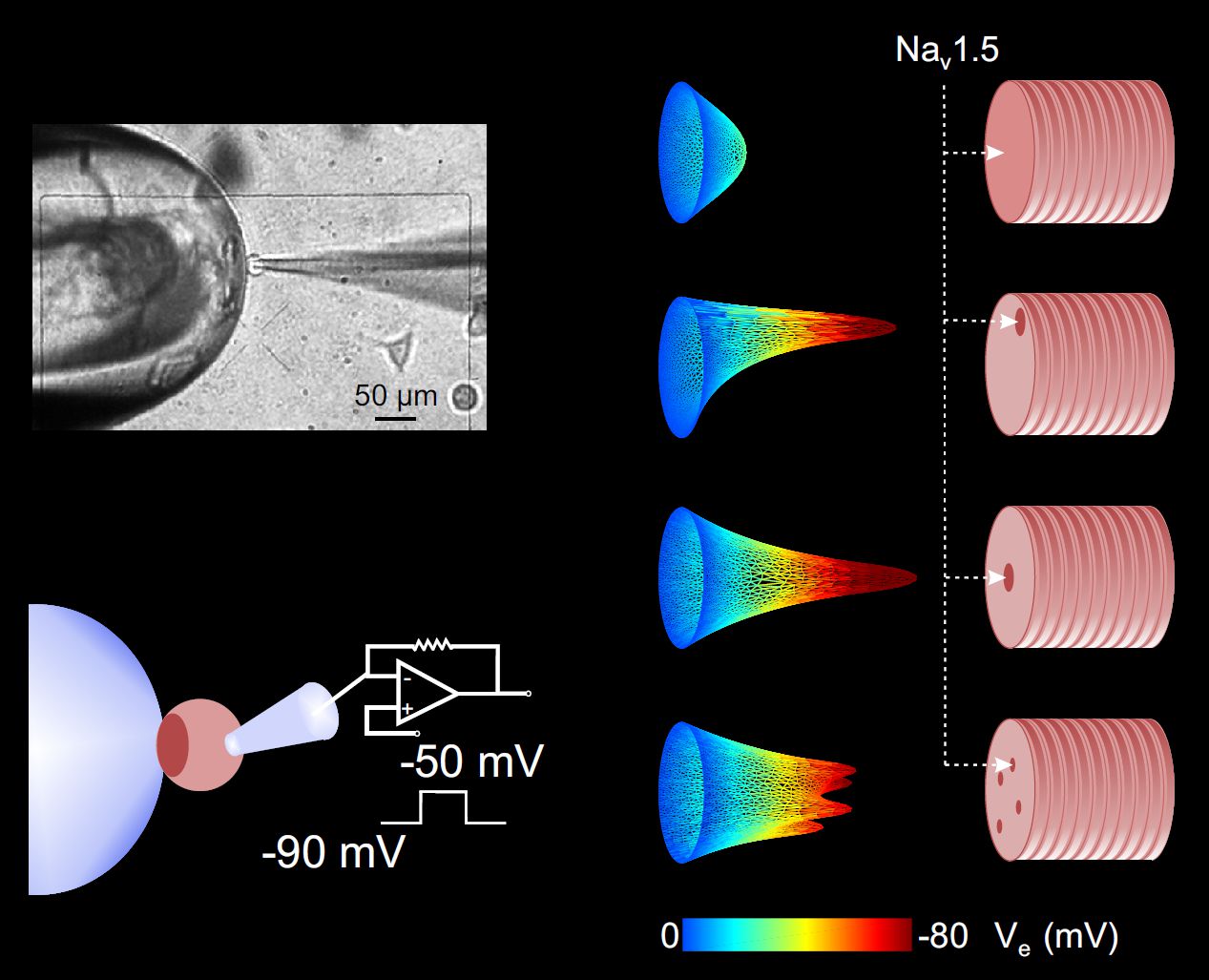
Distribution of cardiac sodium channels in clusters potentiates ephaptic interactions in the intercalated disc. J. Physiol. 596:563-589, 2018 DOI pdf
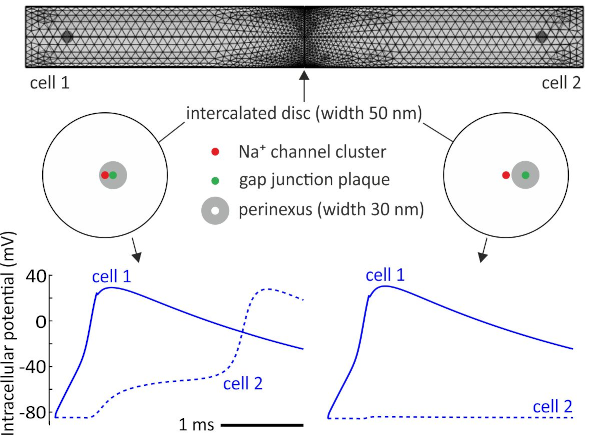
Localization of Na+ channel clusters in narrowed perinexi of gap junctions enhances cardiac impulse transmission via ephaptic coupling: a model study. J. Physiol. 1-33, 2021 DOI pdf
Characterization of conduction at the cellular level in cardiac tissue consisting of heterogeneous cell populations
The myocardium is known to become heterogeneous in the diseased heart, and myocardial heterogeneity is known to be a substrate of conduction disturbances. Among these disturbances, slow conduction and conduction block are the typical mechanisms leading to reentrant arrhythmias such as tachycardias and fibrillation. Slow conduction and conduction block can result from a reduction of inward membrane currents or a reduction of gap junctional coupling. However, slow conduction and conduction block can also arise from the presence of heterogeneous cellular phenotypes in cardiac tissue and from the particular arrangement of cells with different phenotypes within the tissue. In bioengineered cardiac strands consisting of mixed populations of cells and in corresponding simulations of cardiac conduction, we are investigating the functional consequences of this cellular heterogeneity. We showed that conduction in strands consisting of mixtures of normal and connexin 43 knock-out myocytes is slow and highly prone to block. In collaboration with Dr. Nina Ullrich (University of Heidelberg), we showed that conduction in strands combining native cardiomyocytes with stem-cell derived cardiomyocytes (as a model of tissue repair) is always impaired in comparison to propagation in strands of native myocytes (as a model of normal tissue). In addition, we replicated using this model the previous observations of the group of Prof. S. Rohr in co-cultures of myocytes and myofibroblasts.
Publications
Nonlinear behaviour of conduction and block in cardiac tissue with heterogeneous expression of connexin 43. J. Mol. Cell. Cardiol. 76: 46-54, 2014 DOI
Slow conduction in mixed cultured strands of primary ventricular cells and stem cell-derived cardiomyocytes. Front. CellDev.Biol. 3:58, 2015 DOI
Myofibroblasts Electrotonically Coupled to Cardiomyocytes Alter Conduction: Insights at the Cellular Level from a Detailed In silico Tissue Structure Model. Front. Physiol 7:496, 2016 DOI
Revisiting cardiac restitution
Action potential duration (APD) and conduction velocity (CV) depend on one or several previous diastolic or interbeat intervals. This dependence, called «restitution», is an important determinant of the stability of conduction and is involved in the generation of arrhythmias. Our goal is to explore restitution by developing new pacing protocols and by exploring novel analyses inspired from systems theory to extract quantitative information regarding restitution and conduction characteristics.
In particular, we developed a framework in which a cardiac cell or cardiac tissue is paced at intervals varying randomly. By considering the investigated cardiac preparation as a system taking an input (the series pacing intervals) and producing an output (i.e., the series of APDs, interbeat intervals), the input-output characteristics of the system can be understood in terms of transfer functions. The identification and the analysis of such transfer functions provide invaluable additional information. This information can be exploited to derive new strategies to quantify the susceptibility to arrhythmia and to identify possible mechanisms. The expected developments may form the basis for a future implementation enhancing clinical electrophysiological testing in cardiac patients, permitting to assist diagnostic and therapeutic decisions.
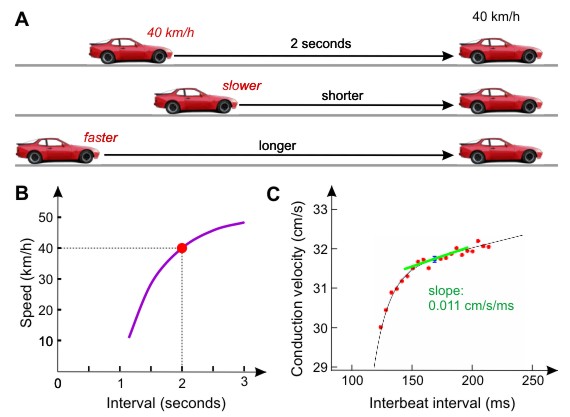
The concept of restitution
A: Drivers learn to maintain an interval of 2 seconds between their own vehicle and the vehicle before them. This interval is stable only if the speed of the follower equals that of the leader. If this interval becomes shorter, the driver of the follower vehicle will slow down. If this interval becomes longer, the driver may accelerate.
B: Representation of this phenomenon as the speed of the follower vehicle vs. the interval between the vehicles, assuming that the leader drives at 40 km/h. Such a representation is called «restitution curve», or «restitution function».
C: Conduction velocity restitution curve of one of our cultured strands of cardiac myocytes. On the road, the behavior of drivers determines the stability and the safety of traffic. Similarly, in cardiac electrophysiology, the form and the slope of restitution curves of conduction velocity and AP duration are important parameters governing arrhythmogenesis.
The transfer functions of cardiac tissue during stochastic pacing. Biophys. J. 96:294-311, 2009 DOI
Alternans resonance and propagation block during supernormal conduction in cardiac tissue with decreased [K+]o. Biophys. J. 98:1129-1138, 2010 DOI
Uncovering the Dynamics of Cardiac Systems Using Stochastic Pacing and Frequency Domain Analyses. PLoS Comput Biol 8:1-14, 2012 DOI
Stochastic pacing reveals the propensity to cardiac action potential alternans and uncovers its underlying dynamics. J. Physiol. Epub, 2016 DOI